December 2020
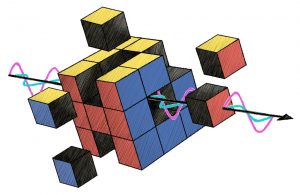
In the trade, we talk a lot about changes in enthalpy, especially when we are looking at total heat exchange over an evaporator. Sometimes, you will bump into the word entropy, and I wanted to take a stab at making it more understandable. Many people understand entropy as the condition in which molecules become more […]
Read more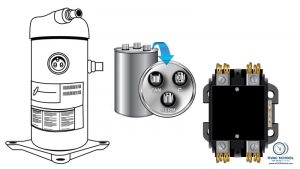
Replacing a compressor is expensive, time-consuming, and physically taxing. If we are replacing a compressor, I want us to be doggone sure we aren't going to be dealing with the same thing again, and this often includes a shiny new contactor and capacitor (on single-phase units). We received a comment recently that called out the […]
Read more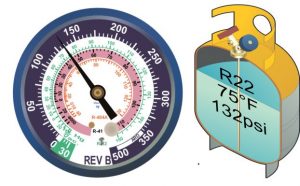
In HVAC systems, liquid and vapor will exist at the same time and place. We call that condition saturation, or we say that the refrigerant is “at saturation.” Phase changes occur in the evaporator and condenser, so these are spots where liquid and vapor coexist while the system is running. Saturated conditions occur whenever liquid […]
Read more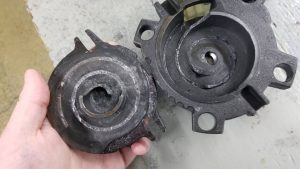
Compressibility is the ability of a substance to be squeezed into a smaller volume. It is the change in volume and increase in density that results from an increase in pressure. The subject of compression should be familiar to HVAC techs. After the return air passes over the boiling refrigerant in the evaporator coils, the […]
Read more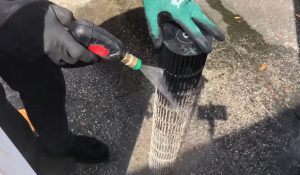
Ductless systems didn’t gain popularity in North America until relatively recently. However, it’s no surprise that they’re becoming quite common. They are quiet, efficient, and well worth the price in the long run. Highwall ductless systems have their downsides, though. When they get dirty, they can spread gunk beneath them and make a room smell […]
Read more